Ocean thermal energy conversion could power the world’s tropical islands, if it ever gets out of the “innovation valley of death.”
This story by Mark Betancourt originally appeared in Eos and is republished here as part of Covering Climate Now, a global journalism collaboration strengthening coverage of the climate story.
Benjamin Martin came to the tiny Japanese island of Kumejima to work as an English teacher after graduating from a business school in landlocked Arizona. Now he runs a power plant fueled by ocean water. “I don’t have an engineering degree, and I do all the maintenance for our electricity,” he said. “It’s relatively easy.”
The plant, which looks like a cross between a lighthouse and a jungle gym, generates a negligible amount of power, only about 100 kilowatts (KW). It was built in 2013 to demonstrate a process called ocean thermal energy conversion, or OTEC. The idea behind OTEC isn’t new, and it’s deceptively simple. Like most power plants, the facility uses vaporized liquid to spin a turbine and generate electricity. The difference is that instead of burning fuel, the plant gets its energy from sun-warmed water from the ocean’s surface. Cold water pumped up from a depth of several hundred meters cools the vapor again, creating a heat engine.
For now, Kumejima depends mostly on diesel fuel, shipped in at a premium, to provide electricity for its 8,000 residents. But residents of the island hope to someday sever that dependency by building a 5-megawatt (MW) OTEC plant that, with a bit of solar, could cover all of its energy demand.
Such an endeavor would be expensive, and the plan is to help pay for it by sharing the cold-water intake pipe with the various cold-water industries already thriving on the island — from prawn farming to a deep-sea water spa, to greenhouses in which the water chills the soil so that it’s the optimum temperature for growing spinach. Cold seawater can even be used for air-conditioning.
“Our research center is cooled by deep-sea water, which reduces the amount of power we need for cooling by about 90 percent,” said Martin, who also serves as secretary general for the Ocean Thermal Energy Association, a group with members from around the globe who want to see OTEC deployed and expanded in the power sector.
On paper, they’re right to be optimistic. The theoretical potential of OTEC is vast. It could produce at least 2,000 gigawatts (GW) globally, rivaling the combined capacity of all the world’s coal power plants on their best day. And unlike many renewables, OTEC is a baseload source, which means it can run 24/7 with no fluctuation in output.
But the conditions necessary to make the process viable — at least a 20-degree Celsius difference between surface and deep water — occur only near the equator, far from most of the world’s power demand and much of its wealth.
That’s why, despite its simplicity and decades of small, successful demonstrations, OTEC has yet to take hold in the renewable power industry. Its high initial capital costs have kept investors away, especially as other renewables like solar and wind get cheaper by the minute.
But places like Kumejima and dozens of small island states, many of them among the poorest countries in the world, could benefit enormously from ocean thermal power. If they can raise the money to get started, energy independence and carbon neutrality may be literally at their doorstep.
“The island hopes to be 100 percent carbon-free by 2040,” Martin said of Kumejima. “But we need OTEC to get there.”
More of a Warmth Engine
The universe runs on contrasts and the forces of entropy. Thermal power, for instance, isn’t generated by heat but by its ability to do work as it cools. Even a modest amount of heat can do the job, as long as there’s a temperature gradient to be exploited.
Most OTEC systems use liquid ammonia, which has a very low boiling point, as what’s called the “working fluid.” Warm water from the ocean surface flows into a heat exchanger, where it causes the ammonia to evaporate. (Other “open” systems use the seawater itself as the working fluid, first exposing it to a vacuum to lower its boiling point.) As the vapor expands, it flows around the blades of a turbine. The vapor then enters another heat exchanger, where cold water pumped up from the deep ocean causes it to condense again. The pressure differential between the two chambers on either side of the turbine pulls vapor from one to the other, spinning the turbine and generating electricity. Some of that electricity is used to run the pumps. What’s left can feed the grid.
At first glance, it looks like a painfully inefficient process; only about 2 to 3 percent of the energy in the seawater is converted to electricity. But the fuel is free and virtually infinite. Output is limited only by how much water you can pump at a time.
“OTEC can be cost-competitive, it’s just no one’s done it yet.” — Benjamin Martin
And there’s the rub. For OTEC to be viable — that is, for it to be competitive with other renewables like wind and solar — the plants need to be huge. To get anywhere near wind’s or solar’s average cost of 0.02–0.06 cents per kilowatt-hour (KWh), an OTEC plant would have to keep the equivalent of four Niagara Falls flowing through its heat exchangers at all times.
A 100–MW OTEC plant (the equivalent of about 500 acres, or 202 hectares, of solar panels) would need a cold-water intake pipe that’s 7–10 meters in diameter to work efficiently. Just to scale up to a modest 1–MW plant, Kumejima expects to spend between $60 million and $80 million on a 1.5–meter pipe.
So far, funding for that kind of leap of faith has proven to be elusive. “OTEC can be cost-competitive,” Martin said. “It’s just no one’s done it yet.”
The Curse of the First
The concept of using warm and cold ocean water to generate electricity is about as old as the generation of electricity itself. French scientist Jacques-Arsène d’Arsonval theorized the process in 1881, just as the notion of using a steam engine to rotate coils of copper wire around a magnet was emerging as a source of commercial and industrial power. His student, Georges Claude, a rebellious and hardheaded entrepreneur who got rich selling neon lights, built the first OTEC plant in Cuba in 1930. At first cockily optimistic, he eventually admitted that running it cost about four times more power than it generated.
And Claude had a lot of trouble with the cold-water pipe. He sank a handful of failures in Matanzas Bay, losing $1 million with each attempt. The one that finally worked was destroyed by a hurricane.
The moral of Claude’s story is that when it comes to big, novel machines, failure is expensive. And before Claude had even begun his less-than-convincing demonstration, coal had become ubiquitous as the fuel for large-scale power plants. Unlike two-temperature seawater, coal (and, later, oil and natural gas) was perniciously easy to ship anywhere in the world.
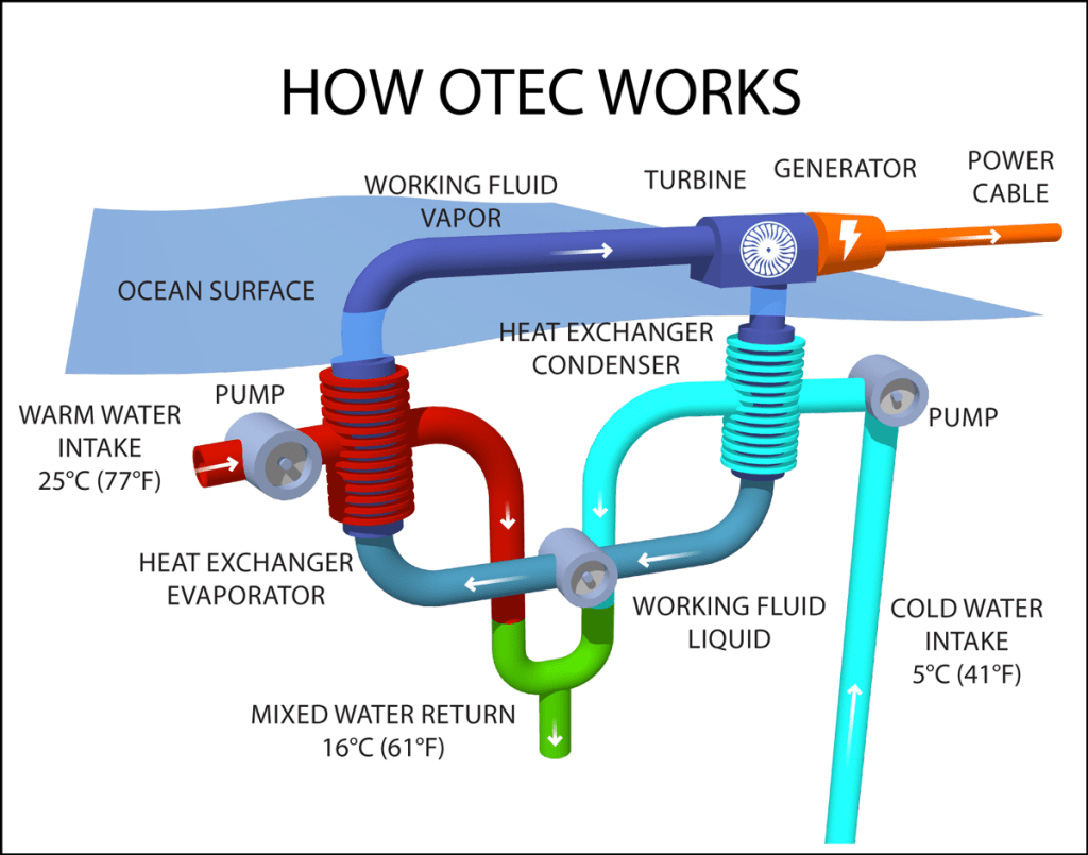
Predictably, global interest in OTEC has followed oil prices. After the crises of the 1970s, President Jimmy Carter signed a bill calling for 10,000 MW of OTEC capacity to be up and running by 1999. Then oil prices stabilized, administrations changed, and other than a few demonstration projects, nothing happened.
OTEC projects began to crop up again after the 2008 financial crisis, coupled with growing concern over climate change. Makai Ocean Engineering, based in Hawaii, was the first to connect an OTEC plant to the US power grid. But like Kumejima’s facility, it was a temporary demonstration, generating only enough electricity to run about 120 homes. The cold-water pipe is still operating, though, allowing a local fishery to grow and sell Maine lobsters.
Plans for a score of small-scale OTEC projects have been announced, including in Bora Bora — where one resort is already using deep-sea water for air-conditioning — China, Martinique, and Puerto Rico. But this time, the meteoric rise of wind and solar power has simply overtaken marginal renewables like OTEC.
The main advantage of wind and solar is their modularity. The technology can be proven at full scale with a single turbine or panel; scaling up is a matter of simply manufacturing more of them. OTEC, on the other hand, requires a huge investment — and the risk that goes with it — before even the first kilowatt is generated.
“The big risk, the big unknown, the big killer for OTEC … is the cold-water pipe.” — George Hagerman, Center for Coastal Physical Oceanography
The potential pitfalls of installing a true commercial OTEC plant can come from unexpected quarters. The Korea Research Institute of Ships and Ocean Engineering plans to install a 1–MW, shore-based plant on Tarawa atoll in Kiribati, smack in the middle of the Pacific. The plant components were successfully tested on a barge off the Korean coast in 2019, generating the largest OTEC current to date — about 338 KW — even in suboptimal conditions. But the project stalled when the COVID-19 pandemic drove up the cost of shipping the components to Kiribati.
George Hagerman, a senior project scientist at Old Dominion University’s Center for Coastal Physical Oceanography, questions whether OTEC itself is quite ready for its moment. The main reason hasn’t changed for nearly a hundred years.
“The big risk, the big unknown, the big killer for OTEC — and it’s what actually destroyed Georges Claude’s plant in Cuba — is the cold-water pipe,” said Hagerman.
The Kilometer-Long Thorn in OTEC’s Side
Most OTEC enthusiasts agree that large, shore-based plants would be too expensive, mostly because the cold-water pipe would have to be too long. Go down about a kilometer pretty much anywhere in the world, and you’ll feel an icy 4 degrees Celsius, but running the pipe from shore adds to that distance and exposes a fixed pipe to the battering of waves and currents.
To keep the pipe shorter and avoid this exposure, the plant could instead be mounted on a barge or platform and parked where a flexible pipe could be suspended straight down to cold water. Power could be run ashore via the same kind of cable currently being used by offshore wind facilities.
There’s still the problem of making the pipe wide enough to efficiently draw massive amounts of water. OTEC believers say that’s mostly a matter of tweaking the designs and materials currently being applied in other industries that move large volumes of water.
But Hagerman, who’s been researching and developing ocean energy technology for 40 years, knows it’s not that easy. Even designs that seem straightforward can run into unexpected and expensive challenges, especially in the chaotic environment of the sea. “When people actually have to build stuff that’s got to survive in the ocean and be insured, costs double. Insurance premiums triple,” he said. “And all of a sudden, what looked good when you announced it, you can’t actually get finance to build.”
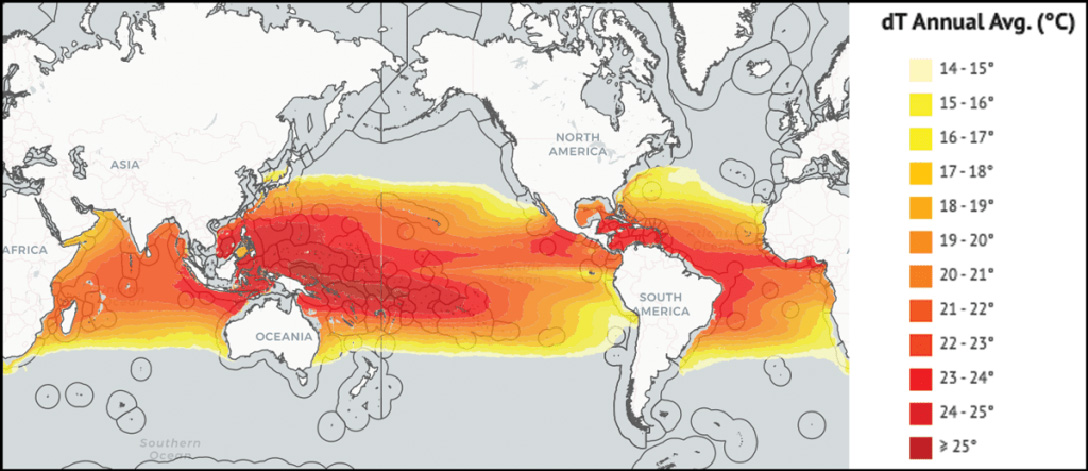
Hagerman thinks it would be better to forgo the suspended intake pipe entirely, replacing it with a tunnel beneath the ocean floor. A tunnel would be easier to design as wide as necessary to make OTEC cost-efficient and could bring cold water ashore to a fixed plant (where it could also feed cost-sharing ventures like aquaculture and air-conditioning) or to a movable barge that could detach from the tunnel and move out to sea in the event of a storm.
“Elon Musk is doing tunnel boring all over the place right now,” said Hagerman, who suggested that OTEC is the perfect project for a climate-conscious billionaire. “You would have basically a permanent cold-water intake conduit on an island or a coastline, and it would be there for the life of the planet.”
According to Hermann Kugeler, Makai’s business development manager, the cold-water pipe is an inherently expensive part of the OTEC system. “We’ve done what we can, but that’s kind of the one cost [for which] we don’t see much more reduction,” he said.
Instead, Makai’s recent focus has been on building cheaper heat exchangers that won’t rust. The relative acidity of deep-sea water is hard on aluminum, which composes most off-the-shelf components, so the second heat exchanger must be made of expensive titanium. Kugeler said that his team has been working out a design that uses much thinner plates to separate the seawater and working fluid and therefore requires less titanium to build. It’s also significantly smaller and lighter, which lowers the cost of mounting it on a platform.
Although a 100–MW plant may still be only theoretically possible, “a commercial scale of 10 MW is probably commercially feasible today using this technology,” said Kugeler.
A Niche Market
That may be enough to sneak OTEC into the commercial space. Dan Grech, the young and exuberant CEO of a UK-based startup called Global OTEC, challenges the idea that an ocean thermal plant has to be enormous to be viable. Instead of making plants bigger, he wants to keep them relatively small and rightsize the market; namely, scattered island nations that don’t need much power but need to spread it over a vast area.
“Our plan is to standardize as much of the OTEC platform as possible so it can be mass-produced for the widest deployment possible,” Grech wrote in an email. He’s worked out that a few dozen small island nations have a combined installed capacity of about 12,000 MW — barely 1 percent of the installed capacity of the United States alone — and most of it is generated by fossil fuels.
“Annually, we spend more than $20 billion a year on oil imports,” said Albert Binger, secretary general of the Small Island Developing States (SIDS) Sustainable Energy and Climate Resilience Organization (SIDS DOCK), a climate- and energy-focused coalition of 32 developing small island states. More than $6 billion of that collective oil is burned for energy. It’s an expense they can little afford. Binger added that many small island nations still haven’t recovered from the debt they incurred during the oil crisis that began in 1979. That’s partly because oil costs more for tiny, remote places; suppliers see modest orders with astronomical shipping costs and raise prices.
And the volatility of oil prices stunts small island nation economies, Binger said, because businesses can’t rely on consistently affordable electricity to produce their goods. “If we [SIDS] had taken a lot of that money and put [it into] OTEC system[s] previously, we would be in a completely different situation,” said Binger. “We need an energy source that is secure, reliable and has a pretty good price.”
Many of the archipelagos that make up island nations are the exposed peaks of submerged mountain ranges of extinct volcanoes called seamounts. Their shorelines are more like cliffsides, and kilometer-deep water can be found within a few kilometers of shore. This bathymetry makes them ripe for OTEC.
“We have more ocean than the EU [European Union] has land,” said Binger of small island nations; in fact, it’s about 16 times more. So by definition, there’s very little building space for land-based renewables. Most of the V-shaped atoll that comprises Kiribati’s capital, for example, is less than a kilometer wide. “Every megawatt of solar that we put up means about 2 acres of land taken out of something or not available for something,” said Binger.
In 2021, SIDS DOCK signed an agreement with Global OTEC to develop ocean thermal power, starting with a small 1.5–MW barge in São Tomé and Príncipe, off the central African coast. The plan is to use that first barge to prove the model, then scale up to a larger one or more barges over the following few years. Like Kiribati, many islands are also considering shore-based plants —the associated secondary cold-water industries like those on Kumejima could bring much-needed jobs. Open OTEC systems, which desalinate seawater as it’s vaporized, could also yield fresh water on islands where it’s becoming increasingly scarce.
And despite their limited resources, all of the SIDS DOCK countries are signatories to the Paris Agreement. That’s ironic given that many of them are net carbon sinks; their ocean water and tropical biomass absorb more carbon dioxide than their human population emits. But, Binger said, island countries are at the forefront of converting to renewable energy out of self-preservation—some could be swallowed entirely by the rising sea if climate change isn’t slowed.
“If you can’t speak up for yourself when you’re vulnerable, you can’t blame anybody else,” he said.
“A Tipping Point”
Researchers at the University of Hawai‘i at Mānoa (UH Mānoa) have been working out the environmental impacts of OTEC should it ever gain a real foothold in the market. One OTEC plant, they found, would have a negligible effect on the physical environment in the ocean around it. But what about thousands of plants all over the world?
On a massive global scale with high plant density, OTEC systems would mix up enough seawater to nullify the thermal gradient that makes the process work. “The core idea is that OTEC is self-limiting,” Gérard Nihous, who recently retired from the University of Hawai‘i, wrote in an email, “and could have, at sufficient scale, unintended and unwelcome consequences.”
The innovation valley of death, or a Catch-22: Investment would almost certainly follow a successful OTEC plant that generates commercial-scale power. But that can’t happen without…investment.
The ocean is vast, however, and there’s room for quite a few OTEC plants. In a 2018 paper, Nihous and two other colleagues from the University of Hawai‘i wrote that as many as 15,000 plants, spaced 30 km apart and within 100 km of land, would avoid any large-scale disruptions (even if they were all plopped down in the ocean at once, which they wouldn’t be). These plants would generate more than 2 terawatts (TW) of electricity, making OTEC a viable way, with other renewables, to help power the world.
Of course, all of that is theoretical. Like Kumejima and every other place looking to take advantage of ocean thermal energy, SIDS DOCK and Global OTEC are still searching for funding to even begin their modest projects. It’s a frustrating Catch-22: Investment would almost certainly follow a successful OTEC plant that generates commercial-scale power, say, 10 MW. A recent report by Ocean Energy Systems, part of the International Energy Agency, suggests that even 2.5 MW would be enough to quell investors’ fears about the cold-water pipe.
But that can’t happen without investment. Entrepreneurs call it the “innovation valley of death.”
There’s a chance OTEC’s ticket out of the valley could be as a complement to its rivals, wind and solar. “As the grid penetration of those increases, there’s going to be a pretty significant need for energy storage or something to provide baseload power, which OTEC does,” said Kugeler. If the cost of storage is factored in, he said, OTEC’s price per kilowatt hour looks competitive.
But it remains to be seen how much global capital will be spared for a technology that not everyone in the world can use.
Tim Ramsey, program manager for marine energy at the Water Power Technologies Office with the US Department of Energy, said that OTEC’s potential may not be realized until it’s free from geographical constraints. That prospect isn’t as far-fetched as it may seem.
“There will just potentially be a tipping point where all of a sudden the economics makes sense and it just blows up and you see it everywhere.”
The same electricity storage technologies being developed to stabilize intermittent renewables like wind and solar could light a fire under OTEC, too. Picture ships shuttling massive charged batteries from remote ocean plants to the mainland; or self-propelled plants (grazing barges) that could hunt out the best temperature gradients and use OTEC power to produce hydrogen fuel, then ship it around the world. That would open up a whole new lane within the energy market, to the benefit especially of developing nations that control the lion’s share of the warm ocean.
Ramsey said that’s part of why OTEC is still on DOE’s radar. “It’s one of those things that there will just potentially be a tipping point where all of a sudden the economics makes sense and it just blows up and you see it everywhere,” he said.
That may be of little comfort to the millions of islanders currently living under the thumb of expensive and destructive diesel power. All the clean energy they need stretches out in every direction, as far as the eye can see. But without investors who are willing to take the plunge, it’s out of reach.
Having watched how seawater has sustained his little island community on Kumejima, Martin feels certain OTEC’s day is coming; people just need to understand it. That’s easy if they can see it work. When local schoolchildren come to tour the 100–KW test plant, he invites them to touch the two intake pipes that snake up from the ocean.
“You feel the cold, you feel the hot,” he said. “It’s simple.”